Research
Our experimental research group explores the physicochemical properties of natural and artificial biomolecular systems and their potential applications in nanotechnology and synthetic biology.
Nucleic acid nanotechnology
DNA and RNA molecules can fold into a variety of different molecular conformations with different mechanical and dynamical properties. For instance, complementary DNA molecules can bind with each other to form relatively rigid double-stranded molecules, while self-complementary sequences can fold back to form hairpin loops. What is important here is that the base sequence of the nucleic acids determines the structure they will adopt. In nucleic acid nanotechnology, we are interested in utilizing such sequence-programmable nucleic acid self-assembly reactions for the creation of artificial molecular nanostructures (including, but not limited to DNA and RNA origami structures), and also for the realization of dynamical devices, molecular machines, computing devices and biosensors. An important trick that is frequently applied in our work are strand displacement (or strand invasion) reactions, in which one DNA or RNA strand can replace another strand within a molecular nanostructure via a so-called branch migration process. This facilitates isothermal, reversible switching of the structure, which is the basis for a plethora of applications in dynamic DNA nanotechnology. One major focus of the group currently is the integration of several functions (such as sensing, computing, and actuation) into a single system with the goal to create molecular-scale equivalents of robotic systems.
Cell-free synthetic biology and synthetic cells
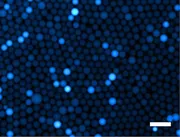
Synthetic biology is often referred to as the engineering of biology and involves the rational design of synthetic biological parts and devices in order to perform useful, technologically motivated tasks. Cell-free synthetic biology is its sub-field that is devoted both to the establishment of biological functions outside of the cellular context as well as to the bottom-up creation of life-like systems. Cell-free biology has the advantage of being released of many of the constraints of living systems, and it also allows an easier combination of biological and non-biological components. In cell-free synthetic biology our group is engaged in a variety of research efforts. One is devoted to the design and study of cell-free gene circuits as components of synthetic cellular systems. In this context, we also work on various approaches to compartmentalize cell-free systems – ranging from emulsion droplets over lipid vesicles, gels and coacervates to chip-based systems. Artificial cells are of interest as cell-sized bioreactors, but also as an attempt to create minimal living systems that will help us understand the transition from non-living to living – from matter to life. Yet another way to look at artificial cells is as cell-scale “soft” robotic systems – ideally, artificial cells will be able to communicate with their environment and perform useful or interesting functions such as computation, production or motion. Our current efforts also involve the generation of multicellular structures composed of communicating artificial cells and the establishment of out-of-equilibrium conditions which are needed to generate non-trivial dynamical behaviors. This also requires the application of various microfluidic and 3D printing techniques.
Gene circuits and in vivo computing

One of the goals of synthetic biology is to program biological systems in order to perform useful tasks. Biology uses DNA to store information and gene regulatory mechanisms to perform computation – thus one approach to program living systems is to alter their genetic make-up and to establish synthetic gene circuitry. In our work we utilize traditional gene regulatory mechanisms involving transcription factors, but also RNA-based components such as riboswitches, synthetic riboregulators, or CRISPR-based mechanisms. We are particularly interested in utilizing concepts from dynamic DNA nanotechnology and DNA computing in the context of living cells. In particular, we explore various ways to apply RNA strand displacement processes for in vivo gene regulation and computation. The resulting synthetic gene circuits may be used to establish control circuits for various cellular processes. For instance, they may be utilized for sensing, computing, decision making and conditional gene expression, or to establish and control intercellular communication, which could have manifold applications ranging from biosensing over metabolic engineering to biomedical applications. Among other topics, we currently focus on the realization of conditional CRISPR mechanisms, and the programming of pattern formation and artificial population dynamics in bacterial cell consortia.